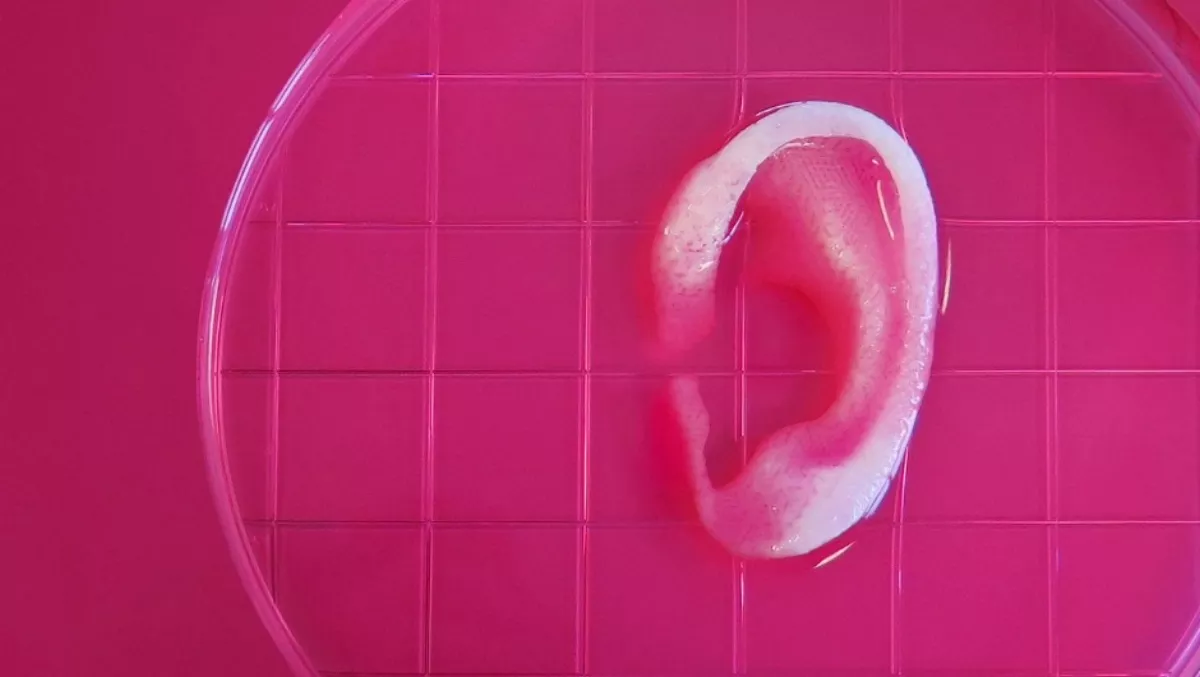
Latest breakthrough in 3D printing: Human ears..?
In another breakthrough using 3D printing, medical researchers in North Carolina have successfully produced muscle, cartilage, and bone templates that can potentially used surgical implants.
Using a sophisticated, custom-designed 3D printer, regenerative medicine scientists at Wake Forest School of Medicine have proved that it is feasible to print living tissue structures to replace injured or diseased tissue in patients.
Reporting in Nature Biotechnology, the scientists said they printed ear, bone and muscle structures, and when implanted in animals, the structures matured into functional tissue and developed a system of blood vessels. Furthermore, these early results indicate that the structures have the right size, strength and function for use in humans.
"This novel tissue and organ printer is an important advance in our quest to make replacement tissue for patients," says Anthony Atala, M.D., Wake Forest Institute for Regenerative Medicine (WFIRM) director and senior author on the study.
"It can fabricate stable, human-scale tissue of any shape. With further development, this technology could potentially be used to print living tissue and organ structures for surgical implantation," he says.
With funding from the Armed Forces Institute of Regenerative Medicine, a federally funded effort to apply regenerative medicine to battlefield injuries, Atala's team aims to implant bioprinted muscle, cartilage and bone in patients in the future.
Tissue engineering is a science that aims to grow replacement tissues and organs in the laboratory to help solve the shortage of donated tissue available for transplants.
According to the researchers, the precision of 3D printing makes it a promising method for replicating the body's complex tissues and organs. However, current printers based on jetting, extrusion and laser-induced forward transfer cannot produce structures with sufficient size or strength to implant in the body.
The Integrated Tissue and Organ Printing System (ITOP), developed over a 10-year period by scientists at the Institute for Regenerative Medicine, overcomes these challenges. The system deposits both bio-degradable, plastic-like materials to form the tissue 'shape' and water-based gels that contain the cells. In addition, a strong, temporary outer structure is formed. The printing process does not harm the cells, the researchers say.
A major challenge of tissue engineering is ensuring that implanted structures live long enough to integrate with the body. The scientists addressed this in two ways. One, they optimised the water-based 'ink' that holds the cells so that it promotes cell health and growth and two, they printed a lattice of micro-channels throughout the structures. These channels allow nutrients and oxygen from the body to diffuse into the structures and keep them live while they develop a system of blood vessels.
It has been previously shown that tissue structures without ready-made blood vessels must be smaller than 200 microns (0.007 inches) for cells to survive. In these studies, a baby-sized ear structure (1.5 inches) survived and showed signs of vascularisation at one and two months after implantation.
"Our results indicate that the bio-ink combination we used, combined with the micro-channels, provides the right environment to keep the cells alive and to support cell and tissue growth," says Atala.
Another advantage of the ITOP system is its ability to use data from CT and MRI scans to 'tailor-make' tissue for patients. For a patient missing an ear, for example, the system could print a matching structure, the researchers say.
Several proof-of-concept experiments demonstrated the capabilities of ITOP. To show that ITOP can generate complex 3D structures, printed, human-sized external ears were implanted under the skin of mice. Two months later, the shape of the implanted ear was well-maintained and cartilage tissue and blood vessels had formed.
To demonstrate the ITOP can generate organised soft tissue structures, printed muscle tissue was implanted in rats. After two weeks, tests confirmed that the muscle was robust enough to maintain its structural characteristics, become vascularised and induce nerve formation.
Furthermore, to show that construction of a human-sized bone structure, jaw bone fragments were printed using human stem cells. The fragments were the size and shape needed for facial reconstruction in humans. To study the maturation of bioprinted bone in the body, printed segments of skull bone were implanted in rats. After five months, the bioprinted structures had formed vascularised bone tissue.
Ongoing studies will measure longer-term outcomes.